Abstract
This article is based on an interview between the two authors.
The human body is like a jungle, with trillions of fascinating things happening inside it every second, most of which occur without our awareness. In this article, we will zoom in on a very interesting part of this jungle of human-body activities—the immune system. The immune system protects the body from intruders with its experts in martial arts, called killer T cells. Killer T cells are responsible for eliminating virus-infected cells so that the virus cannot reproduce itself and spread throughout the body. Join me for an adventurous journey into the immune system, in which we will find out how killer T cells know which cells to attack and which to leave alone.
Professor Peter Doherty won the Nobel Prize in Physiology or Medicine in 1996, jointly with Prof. Rolf Zinkernagel, for their discoveries concerning the specificity of cell-mediated immune defense.
The Body’s Guardian Cells
The immune system is one of the body’s vital systems. It is made up of white blood cells and the substances they produce. The immune system is responsible for keeping us healthy by successfully fighting off infections (To learn more about the immune system, see the Additional Materials.). Viruses are one type of intruder that can invade the body and make people sick. Viruses are tiny particles that infect the cells of an organism, which is called the host. Viruses “trick” the host cells into making more copies of the virus. The infected cells usually die, releasing many newly made viruses that can spread throughout the body and infect more cells (To learn more about viruses, see this Nobel Collection article.).
The immune system has two main ways of dealing with viral infections. One strategy involves the production of antibodies [1]. Certain lymphocytes, called B cells, are responsible for antibody production. Antibodies bind to the virus and inactivate it before it can infect additional cells (Figure 1A). You can think of antibodies like a bunch of football players jumping on the opponent runner (the virus) to prevent the opponent from advancing with the ball.
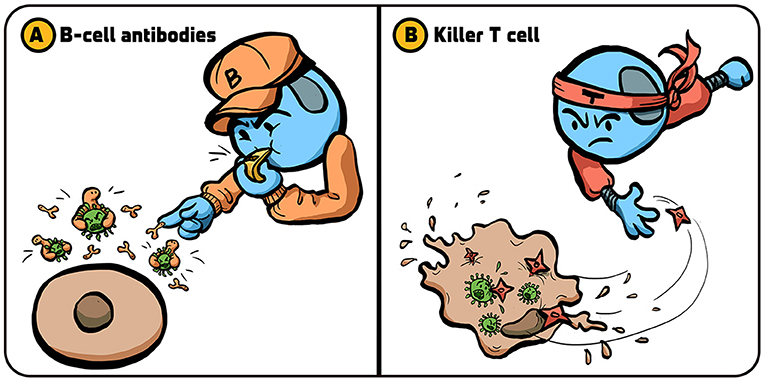
- Figure 1 - Strategies used by the immune system to fight viruses.
- The immune system has several strategies for dealing with viral infections. (A) B cells release proteins called antibodies (small “pliers”) that attach to viruses (green) and prevent them from penetrating into the body’s cells. (B) Killer T cells kill cells that are infected by viruses, preventing the production of new viruses that can infect additional cells. Illustration by: Iris Gat.
As another strategy, the immune system also kills cells that are infected with viruses (Figure 1B). This prevents infected cells from producing many copies of the virus. The lymphocytes that kill virus-infected cells are called cytotoxic T lymphocytes, or killer T cells for short [2]. When killer T cells encounter a virus-infected cell, they kill it by making channels in the cell membrane and triggering the infected cell to self-destruct, through a process called apoptosis (To learn more about apoptosis, read this article.).
Is It Me or Is It Not Me?
The immune system uses extreme measures, like killing cells, to deal with potential hazards. How does the immune system know which structures to make antibodies against or which cells to kill? In other words, how does it eliminate infections without harming the body itself? Research has shown that this is a fine balance, and at the heart of it lies a fundamental question: what is me, or “self” (my own cells and molecules) vs. not-me, or “non-self” (intruders)? It is critical that the immune system answers this question correctly. If, on the one hand, the immune system does not recognize intruders well-enough, then the host remains unprotected against those threats. On the other hand, if the immune system mistakenly recognizes the body’s own cells as intruders, it might attack the body and cause serious damage. Diseases resulting from a misguided immune system attacking the body are called autoimmune diseases, and many of them do not have effective treatments (Researchers are currently checking the possibility that the SARS-CoV-2 virus causing the COVID disease is triggering some rare form of autoimmunity in infected people. Such autoimmunity could be one possible cause of the long-COVID manifestations we are seeing.).
Two Receptors Versus One?
When I started working on killer T cells in the early 1970s, scientists did not yet understand how these lymphocytes could tell “self” from “non-self.” After we discovered that killer T cells are in some way targeting “self” molecules (the transplant proteins recognized in organ graft rejections), most scientists thought that killer T cells had two different types of receptors (proteins that recognize specific molecules) on their surfaces: one type of receptor that could recognize “self” molecule and another type that could recognize foreign (virus), “non-self” molecules (Figure 2A). The idea was that that “self” molecules and “non-self” molecules are two distinctly different types of elements in the body, that require two different types of receptors to be recognized.
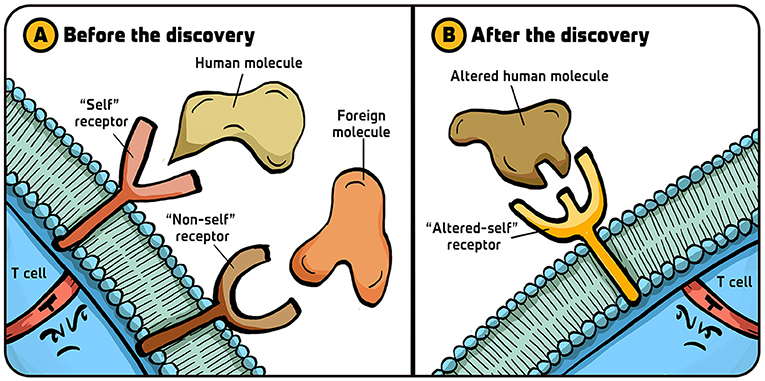
- Figure 2 - Killer T-cell receptor hypotheses.
- (A) Before our Nobel discovery linking killer T cell recognition to “self,” the view that dominated the thinking of those working with “helper” CD4+T cells was that these lymphocytes have two types of receptors on their surfaces—one that recognizes the body’s molecules (“self”) and another that recognizes foreign molecules (“non-self”). (B) After our discovery, it eventually became clear that all T cells have only one immunologically specific receptor, which recognizes changes in the body’s molecules (“altered-self”). Illustration by: Iris Gat.
My colleague Rolf Zinkernagel and I argued that this was not the case—there is actually only one type of receptor on the killer T cell changes in “self” molecules (Figure 2B). This idea allowed a different interpretation of immunology which, as the technical approaches to answering the question improved, turned out to be right. Before I tell you how the recognition of dangerous invaders happens using only one receptor, let me tell you about some of the milestones that led us to this finding.
My Personal Journey: From Feeding the World to Deciphering Immune Secrets
When I was 17 years old, I decided to study veterinary medicine. It was a strange decision because I was a city kid in Queensland, Australia. But I thought that learning veterinary medicine would be a great adventure, and that I could contribute to feeding the world by improving animal production. Today, due to climate change, we know that we will be better off, at least in the advanced countries, by focusing on plant products, but back then we had different ideas. During my Ph.D. studies, I became very interested in how infections cause diseases. So, I went on to study infections in sheep and cattle.
One of my early discoveries was made when I was studying a virus infection of the brains of sheep. At that time, scientists were just starting to understand the role of lymphocytes, but they did not yet understand the specific mechanisms by which those cells operate. In Edinburgh, Hugh Reid and I showed that antibody-producing B cells were present in the brains of the virus-infected sheep, and that those B cells were producing antibodies to fight the virus [3]. This was the first direct evidence that antibody-producing cells could be found in an infected tissue. This discovery inspired me very much, and I wanted to know more about what immune cells were doing in tissues.
While working on sheep in Edinburgh, I realized I need to know more about T cells, and arranged to spend a couple of years at the Australian National University in Canberra where there was a very active group of researchers in this area. The experimental system I chose was lymphocytic choriomeningitis (LCMV) infection of mice. From earlier studies, I adapted a technique to tap the cerebrospinal fluid compartment that bathes the brain to obtain the inflammatory WBCs, which were largely T cells that invade the brain and its surrounding membranes (the meninges) following LCMV infection. Then, a medical graduate from Basel University named Rolf Zinkernagel arrived in the lab. Together, we conducted the experiments that, some 20 year later, won us the Nobel Prize in Physiology or Medicine.
One Receptor Recognizing Altered-Self
Rolf and I did the following experiment: we infected laboratory mice with LCMV and, after a week (at the peak of their immune response), we collected the killer T cells the mice produced against LCMV (Figure 3). We placed these killer T cells in little tubes containing LCMV-infected mouse cells. The killer T cells came from LCMV-infected mouse strains that did, or did not, share the same transplantation molecules (called H2 in mice, HLA in humans) as the LCMV-infected ‘target’ cells. We found that the H2 identical, LCMV-immune T cells did a fantastic job of killing the H2 identical targets, while the H2 different killers totally ignored them. Based on these results, we hypothesized that a killer T cell has a single receptor that recognizes altered-self (infected cells) and developed a new theory of T cell immunology around that.
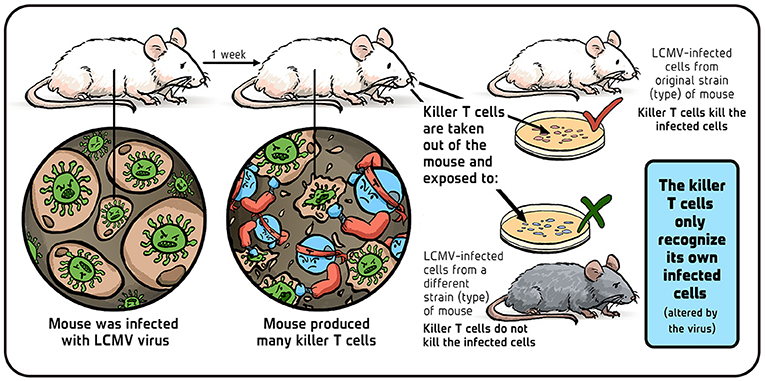
- Figure 3 - Do Killer T cells attack cells from different mouse strains? Illustration by: Iris Gat.
In other words, we assumed that the virus changes one of the normal self-molecules—molecules of non-infected cells, which are similar in genetically identical mice and different in genetically different mice. These self-molecules are always present on the surface of host cells and we figured that killer T cells spot this virus-induced change as a signal of infection and respond by killing the infected cell [4].
At that time, we did not have the experimental techniques to prove that our hypothesis was correct. But technological advances that happened in the next two decades allowed others to prove that we were right [5–8]. Using these new techniques, self- molecules called major histocompatibility complex (MHC) molecules were found on the surface of cells. When a virus invades a cell, the viral proteins get chopped up, and small virus fragments are displayed on the surface of the cell, “held” by MHC molecules (Figure 4). Holding the piece of virus changes the shape of the MHC molecules, and this change in shape is what the killer T cells “see” and react to [9]. In other words, T cells recognize an infected, “altered-self” cell by the modified structure of its MHC molecules.
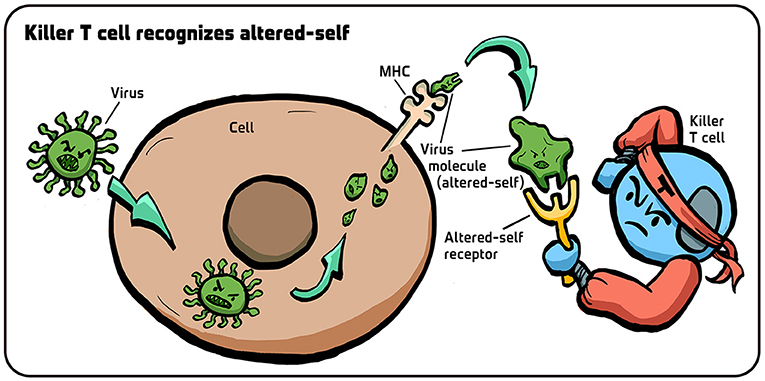
- Figure 4 - How do killer t cells recognize “altered-self”?
- When a virus infects a cell, its proteins are chopped up. Small pieces of the virus (small green structures) are then brought to the surface of the cell, held by MHC molecules. The presence of these virus chunks on MHC molecules changes the structure of the MHC molecule, and the receptors on killer T cells recognize this shape change ("altered-self”), thereby realizing that the cell is infected. Illustration by: Iris Gat.
When our theory proved to be correct, it caused a fundamental shift in our understanding of how T cells—one of the most important parts of the immune system—operate.
Can Our Findings Improve Human Health?
Our understanding of killer T cells could be helpful in several areas of medicine. First, this knowledge could help us to improve vaccines. Now that we know that the immune system recognizes pieces of viruses “held” by MHC molecules, we can hopefully make better vaccines by including a wide variety of pieces from the virus. The more different pieces a vaccine contains, the easier it is to trigger a strong killer T-cell response. COVID-19 vaccines, for example, only contain pieces of one protein (called the spike protein), while the virus has more than 20 different proteins that could potentially trigger killer T-cell responses.
I also focused a lot of my effort on understanding the nature of the protective, ‘resting’ memory T cells that are ‘recalled’ to being killer T cells on reinfection. Others worked out how to ‘wake up’ killer T cells that had localized to cancers but had apparently ‘gone to sleep’ (This is how certain anti-cancer treatments work, which you can learn more about here [10]). Other possible uses for this knowledge could involve new treatments for autoimmune diseases, in which we might find ways to restore balance to T cells and B cells that have gone astray and are attacking the body’s own cells.
Recommendations for Young Minds
I think that scientific training is quite useful to have, even if you do not eventually become an active scientist. The reason is that, as a trained scientist, you learn how to think through a problem (Figure 5), how to deal with data, how to formulate an argument, and how to clearly write and convey your thoughts and ideas. These are important skills that can be helpful in many situations in life.
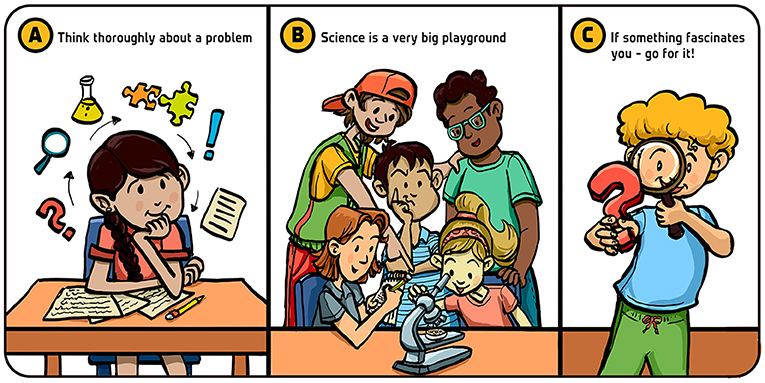
- Figure 5 - Three recommendations for Young Minds.
- (A) Think thoroughly about a problem. (B) Science is a very big playground. (C) If something fascinates you - go for it! Illustration by: Iris Gat.
If you do wish to become a scientist, and specifically a biologist, then I have some good news for you. First, there is still much more to discover in this field. Second, biology— especially biomedical research—offers great opportunities for many types of people with various skill sets and approaches. Some might have computer skills and want to set up systems that analyze and link data. Others might like to perform thought experiments and formulate new ideas and theories. People with good management or product-development skills can also flourish in biomedical research. It is a very big “playground” that holds space for many types of people. Those of you who enjoy connecting the dots in some way might especially enjoy a career in science.
The last thing I will tell you is: if something fascinates you—go for it! Dive into it as deeply as you can and do everything possible to pursue it. You will never know where your interests might lead you. During this voyage, you will gain unique ways of thinking that will serve you wherever you end up. So, instead of being discouraged by the uncertainty that life brings, be confident in your skills and trust that you will get a chance to use them in exciting ways.
Additional Materials
- How the Innate Immune System Fights for Your Health
- The Immune System, in Sickness & in Health—Part 1: Microbes and Vaccines
- Types of immune responses: Innate and adaptive, humoral vs. cell-mediated | NCLEX-RN | Khan Academy
- Doherty, P. (2006). The Beginner’s Guide to Winning the Nobel Prize: Advice for Young Scientists. Columbia University Press.
Glossary
Immune System: ↑ The defense system of the body that protects us against infections and certain diseases.
White Blood Cells: ↑ the main players in the immune system that help the body fight infections.
Antibodies: ↑ Proteins that attach to viruses and inactivate them.
Lymphocytes: ↑ White blood cells responsible for producing antibodies and killing infected cells. B cells and T cells are lymphocytes.
Cytotoxic T Lymphocytes: ↑ Also called killer T cells; white blood cells that kill virus- infected cells.
Apoptosis: ↑ A “suicide” process of cells that gets rid of damaged or infected cells.
Autoimmune Diseases: ↑ Diseases where the body is being attacked by its own immune system.
Receptor: ↑ A protein on the surface of a cell that binds to a specific molecule that matches its shape, triggering a cellular response.
Immunology: ↑ The study of how the immune system functions.
Major Histocompatibility Complex (MHC): ↑ Self-molecules found on the surface of cells.
Acknowledgments
We wish to thank Prof. Steffen Jung for his valuable comments on the manuscript, Iris Gat for providing the figures, and Susan Debad for copyediting the manuscript.
Conflict of Interest
The author NS declared that they were an employee of Frontiers, at the time of submission. This had no impact on the peer review process and the final decision.
The remaining author declares that the research was conducted in the absence of any commercial or financial relationships that could be construed as a potential conflict of interest.
References
[1] ↑ Johnson, A., Lewis, J., Raff, M., Roberts, K., and Walter, P. 2002. Molecular Biology of the Cell. New York, NY: Garland Science p. 4.
[2] ↑ Andersen, M. H., Schrama, D., thor Straten, P., and Becker, J. C. 2006. Cytotoxic T cells. J. Investig. Dermatol. 126:32–41. doi: 10.1038/sj.jid.5700001
[3] ↑ Doherty, P. C. 1970. Studies in the experimental pathology of louping-ill encephalitis (Doctoral dissertation). Edinburgh: University of Edinburgh.
[4] ↑ Zinkernagel, R. M., and Doherty, P. C. 1979. MHC-restricted cytotoxic T cells: studies on the biological role of polymorphic major transplantation antigens determining T-cell restriction-specificity, function, and responsiveness. Adv. Immunol. 27:51–177. doi: 10.1016/S0065-2776(08)60262-X
[5] ↑ Townsend, A. R. M., Rothbard, J., Gotch, F. M., Bahadur, G., Wraith, D., and McMichael, A. J. 1986. The epitopes of influenza nucleoprotein recognized by cytotoxic T lymphocytes can be defined with short synthetic peptides. Cell 44:959–68. doi: 10.1016/0092-8674(86)90019-X
[6] ↑ Brown, J. H., Jardetzky, T., Saper, M. A., Samraoui, B., Bjorkman, P. J., and Wiley, D. C. 1988. A hypothetical model of the foreign antigen binding site of class II histocompatibility molecules. Nature 332:845–50. doi: 10.1038/332845a0
[7] ↑ Garcia, K. C., Degano, M., Stanfield, R. L., Brunmark, A., Jackson, M. R., Peterson, P. A., et al. 1996. An αβ T cell receptor structure at 2.5 Å and its orientation in the TCR-MHC complex. Science 274:209–19. doi: 10.1126/science.274.5285.209
[8] ↑ Garboczi, D. N., Ghosh, P., Utz, U., Fan, Q. R., Biddison, W. E., and Wiley, D. C. 1996. Structure of the complex between human T-cell receptor, viral peptide and HLA-A2. Nature 384:134–41. doi: 10.1038/384134a0
[9] ↑ Wieczorek, M., Abualrous, E. T., Sticht, J., Álvaro-Benito, M., Stolzenberg, S., Noé, F., et al. 2017. Major histocompatibility complex (MHC) class I and MHC class II proteins: conformational plasticity in antigen presentation. Front. Immunol. 8:292. doi: 10.3389/fimmu.2017.00292
[10] ↑ Leach, D. R., Krummel, M. F., and Allison, J. P. 1996. Enhancement of antitumor immunity by CTLA-4 blockade. Science 271:1734–6. doi: 10.1126/science.271.5256.1734